
Advancing Options for Management and Mitigation of
Spray Drift
Risk Mitigation Measures in Europe to Prevent Plant Protection Product losses to the Environment
Spray Drift
Background
Spray drift is defined as the transfer of small spray droplets out of the target area due to wind, exacerbated by poor application practices or sub-optimal application conditions. During application using boom sprayers, the diluted product solution is forced under pressure through nozzles that form spray droplets to provide good product coverage on target surfaces. A range of droplet sizes are generated by many nozzle types with smaller droplets being more vulnerable to drift carried with the prevailing wind. Consequently, one important strategy to reduce drift at the point of application involves reducing or avoiding generation of smaller droplets through choice of nozzle and care and attention taken with application pressure. However, while the droplet size distribution is a major factor influencing the risk from boom sprayers, it is not the only important factor. Therefore, assessments of drift risk cannot be based on the droplet size distribution alone. Other important factors include droplet velocity (speed and direction), droplet structure (the presence of air-inclusions within droplets), and spray structure (entrained air, fan/cone angle, effective porosity).
A range of factors combine at the point of application to dictate spray drift vulnerability. These include the choice and set-up of application machinery, environmental conditions, proximity to and character of the downwind non-target landscape. A general illustration of the potential significance of individual factors in defining drift vulnerability at the point of application is provided below.
Successful drift management/mitigation strategies must be both practical and effective. The ability to manage or control vulnerability considering each of these factors is summarised below.


Application technology choices feature prominently as controllable features in effective drift management strategies. Well-maintained equipment will apply product treatments at the prescribed rate optimizing ability to control product delivery while reducing application errors and managing drift. Further details on the general importance of correct machinery calibration can be found here:
Influences and control
Influences and control
Application technology
Guidelines on Good Practice for Ground
Application of Pesticides.
Food and Agriculture Organization
of the United Nations
Rome, 2001
Basic choices at the point of application such as boom height and application speed also have a role to play. A number of studies relating to the risk of drift from agricultural crop sprayers have identified boom height as one of the most important variables that must be controlled if the risk of drift is to be minimised. Timeliness is a key feature influencing the efficacy of plant protection products and this is constrained by the rate at which product can be applied (also known as “work rate”) and the range of conditions in which a machine can operate. For a boom sprayer, work rate is a function of spraying speed, boom width, application volume and filling/loading time. Ideally, to reduce drift potential applications should be carried out at or below 500 mm above the target surface when using 110° flat fan nozzles that are commonly available in Europe. However, trends towards the use of wider booms and higher spraying speeds to achieve higher work rates can make operations within this range difficult to achieve. Boom suspension design and the use of automated boom height control systems improve the opportunities for operating machines with greater control of boom heights but there are likely to be conditions, particularly involving undulating terrain, when maintaining the correct boom height for spray drift control is very difficult. The risk of drift can also be reduced by reducing spraying speed. With a conventional sprayer fitted with a pressure control system, operating at a slower speed reduces the nozzle pressure as well as the air flow around the spray. Ability to control drift recognising trends for increased work rates is a basis for highly relevant research to design improved drift management and drift mitigation strategies. For further information and examples see UK Defra research project PS2020.
Choice of nozzle also plays a large role in potential management strategies for drift. For boom sprayers operating over field crops, sprays are commonly generated using one of the following nozzle types:
-
Conventional hydraulic pressure flat fan nozzles in which liquid is forced under pressure through an elliptical orifice and the liquid leaving the orifice spreads out into a thin sheet that breaks up into different-sized droplets;
-
Pre-orifice flat fan nozzles in which two orifices are used within the nozzle body – an initial, usually circular, orifice to control flow rate with the final orifice similar to that in a conventional design but with a larger size producing larger droplets;
-
Air-induction flat fan nozzles that again use two orifices but with an air inlet between the two such that air is drawn into the nozzle body by a Venturi action – the outlet orifice is again relatively large producing large droplets that usually have air-inclusions within the droplets (see figure):this changes spray and droplet behaviour considerably.
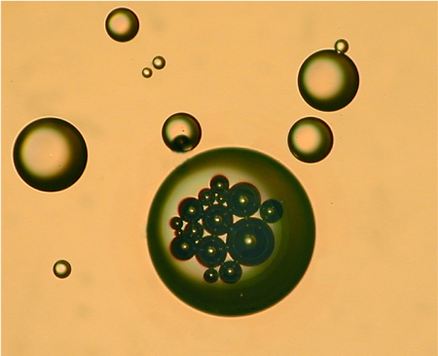
The mean droplet size produced by a nozzle is a function of the nozzle size, spray fan angle and operating pressure.For conventional hydraulic pressure flat fan nozzles, the following rules generally apply:
-
Smaller nozzle sizes having lower flow rates produce smaller droplets and hence finer sprays;
-
Wider spray fan angles give smaller droplets and finer sprays;
-
Higher pressures also give smaller droplets and finer sprays.
It should be noted that the above rules do not apply to many air-induction nozzle ranges particularly in relation to the effect of nozzle size (flow rate) on droplet sizes.Nozzles with the same nominal specification from different manufacturers can have different operating characteristics and this is particularly the case for air-induction nozzle designs.
Classification systems
Classification systems have been devised and promoted by BCPC in the UK and ASABE in the USA for defining the droplet size produced by a nozzle operating at a set pressure in terms of a spray quality.This approach was adopted because measurements of droplet sizes gave different numerical values depending on the measurement method and sampling regime used.The boundaries between spray quality classes are defined by reference nozzles as shown in the table below.

It should be noted that the classification systems do not strictly apply to nozzles creating sprays with air-included droplets such as air-induction or twin-fluid nozzles. However, many information sources will quote a spray quality for such air-included sprays for comparison purposes based on the outer diameter of droplets in the spray.
An example of nozzle performance data including spray quality classification is given below.

Note: air-induction nozzles not given a spray quality classification
Environmental conditions play a potentially large role in defining drift vulnerability. These influences cannot be controlled, but act as constraints or restrictions on application – most notably good practice wind speed recommendations. Higher wind speeds enhance drift transport and good application practice frameworks in place in many Member States accordingly recommend restricting applications to minimize drift potential (e.g. UK Code of Practice recommends applications at 3.2-6.5 km/h (0.89-1.8 m/s measured at boom height) as “ideal” with spraying between 6.5-9.6 km/h (1.8-2.7 m/s measured at boom height) as requiring “special care”. Applications above 9.6 km/h (2.7 m/s measured at boom height) are “inadvisable”) (more information here).
While wind and wind speed are important factors leading to movement from the point of application to non-target areas the role of vertical air movement is often overlooked. Under certain circumstances air stability phenomena can lead to prolonged suspension of droplets in the air column leading to greater transport potential. For example, little vertical mixing may arise when temperature inversions arise (when cool air near the soil surface is trapped below a layer of warmer air). Small droplets may be suspended and move greater distances with only a gentle breeze. Note that the UK Code or Practice acknowledges some air stability effects and recommends that when wind speeds are <2 km/h (0.56 m/s) only medium or coarse spray quality is employed for application.
Relative humidity and temperature effects are also noted. Arable boom applications in Europe may occur under a wide range of conditions ranging from colder late autumn/early spring applications of herbicides in northern Europe to summer applications of insecticides in southern Europe. Higher temperatures enhance drift through increased rate of evaporation of droplets (thereby decreasing droplet size) and thermal effects that may enhance transport. Such vulnerabilities are then extended when the impact of relative humidity is taken into account. Application conditions characterised by less than 50% relative humidity warrant special attention due to increased vulnerability of formation of smaller droplets.
Environmental conditions
Agricultural landscape
The character of the application landscape helps define vulnerability and this includes both the in-crop and off-crop considerations. In-crop, drift vulnerability is influenced by crop development. It is noted that the height of the boom above the ground is greater when operating over tall crops. As noted earlier, greater boom height increases drift potential by allowing greater droplet dispersion in the air column between the nozzle and the application interface (crop or soil surface). The morphological effect of crop type is more complex and subtle - thus it is difficult to generalise regarding impact on drift vulnerability.
Environmental risk assessments may offer recommendations for drift mitigation measures in the form of in-crop and off-crop buffers to protect sensitive non-target areas. The motivation for implementation of such features or strategies is not limited to management of drift issues but could be driven by the needs to manage other risk drivers (e.g. for example management of run-off via use of vegetated filter strips act as a complementary drift mitigation feature). The role and options for implementation of flexible and complementary in-crop and off-crop mitigation was the subject of a preceding SETAC workshop (SETAC MAgPIE, 2016).
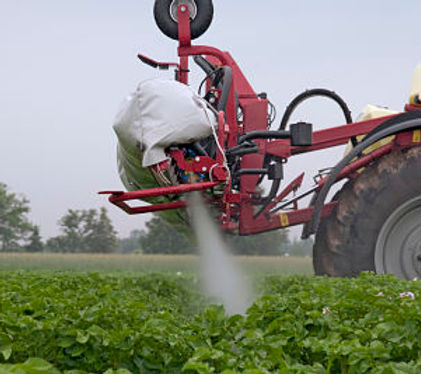